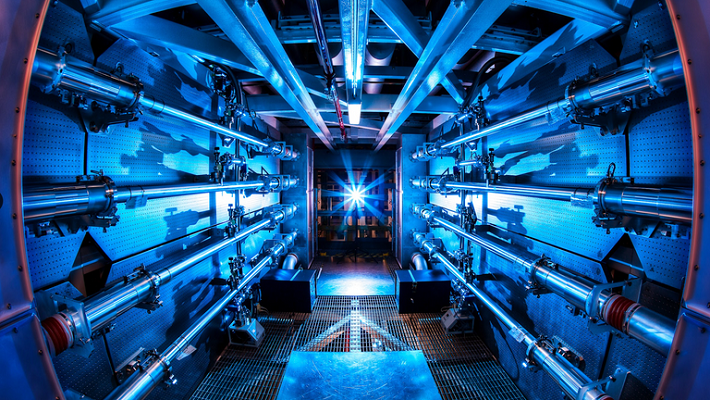
The National Ignition Facility at the Lawrence Livermore National Laboratory in California recently reported that by directing lasers at hydrogen atoms, it was possible to obtain net energy. The lasers produced 3.15 megajoules of fusion energy from 2.05 megajoules of energy they originally produced, which is an increase of around 1.5 times.
This is not the first time that nuclear fusions have given a “net positive energy output”. For instance, the EAST reactor in China, which operated for 17 minutes at a temperature of 126 million degrees Fahrenheit—roughly five times hotter than the Sun—broke the record for the longest sustained nuclear fusion in early 2022.
The Joint European Torus Laboratory (JET) in the UK stated that it has produced 59 megajoules of sustained fusion energy, beating the previous record by two times. This achievement provides a significant boost for the ITER project's collaborators.
These recent developments have undoubtedly made significant progress, but does this indicate that fusion energy is ready for commercial applications?
No. The most recent development is known by scientists as "scientific net energy gain," which denotes that more energy has been released from the reaction than was supplied by the laser.
That is a significant accomplishment that has never been made. However, on a micro level, there is merely a net gain in energy. According to Troy Carter, a plasma scientist at the University of California, Los Angeles,”the lasers utilized at the Livermore facility are only around 1% effective.” This means that the energy required to operate the lasers is approximately 100 times greater than the energy that the hydrogen atoms can eventually get from them.
Therefore, it will still be necessary for researchers to find the "engineering net energy gain," or the point at which the entire process consumes less energy than the reaction produces.
Additionally, they must figure out how to convert the output energy, which is now in the form of kinetic energy from the neutron and helium nucleus, into a form that can be used to generate electricity. By turning it to heat and then heating steam to operate a turbine and power a generator, they could accomplish that. The effectiveness of the procedure is similarly constrained.
All that means that the energy gain will probably need to be pushed much, much higher for fusion to actually be commercially viable.
No doubt there are complex challenges that can push commercial viability of nuclear fusion a light year away from mankind. In this article let's look into the key challenges in the path to attaining "engineering net energy gain".
Varying reactor types
Different approaches to overcome the frightening technical obstacles of fusion are reflected in the many reactor models. Tokamaks, a type of magnetic confinement reactor, are designed to maintain the plasma blazing continuously for extended periods of time (ITER is designed to achieve this for up to 400 seconds). Tokamaks have yet to produce a net energy gain from their plasmas, although getting closer all the time.
The NIF reactor, on the other hand, works to test thermonuclear explosions for military objectives and produces bursts of energy by rapidly burning one tiny bit of fuel after another. To continue a reaction for more than the smallest fraction of a second, however, scientists must swiftly replenish the individual pellets of this fuel, which they have not yet discovered how to do.
"That is very, very tough," said Yves Martin, the deputy director of the Swiss Plasma Center at the École polytechnique fédérale de Lausanne in Switzerland, to Live Science. "You would have to place your next pellet at the period that the cloud increases in the vessel." This pellet, which has a normal diameter of one millimeter, must be placed in a space with a nine-meter width. It still costs several tens of thousands of dollars, as far as I'm aware. It has to drop below one dollar or much less to be engaging.
Expensive isotope
Another problem for fusion reactors is the depletion of tritium, a crucial isotope required in combination with deuterium to power the process. At $30,000 a gram, tritium is among the most costly materials on the planet.
Tritium has a 12.3-year half-life, which means that a large portion of its current stock is already close to becoming useless. Tritium was previously a frequent and unwanted byproduct of nuclear weapons tests conducted outdoors and nuclear fission, which splits atoms rather than combines them and produces a great deal more radioactive waste.
Other ways of producing tritium have been suggested by physicists, such as growing it within nuclear reactors that catch stray neutrons. However, save from a few smaller-scale tests, plans to test tritium breeding at ITER had to be abandoned due to constantly rising costs.
Looking Ahead
Even yet, some difficult problems might take years or even decades to solve. Major advancements in fusion technology have been coming in a constant stream in recent years.
Among them are the successful test of artificial intelligence (AI) in controlling the plasma inside a tokamak, a slew of records in power generation, plasma burn time, and reactor temperatures across multiple experiments, and the rewriting of a fundamental rule that could allow reactors to produce twice as much power.
These developments have led fusion researchers to assert that various techniques are required for a long-term solution to the climate catastrophe and that fusion will play a crucial role in a future carbon-free energy system.